Enzymes achieve catalysis due to the active site cleft present in proteins.
The most important aspect of catalysis is species proximity – the closer the species are to one another, the better.
The rate of reaction of two species can be given by the equation:
rate = k[A].[B]
Typically, reagents without an enzyme have a nanomolar concentration and small rate. When together, however, the enzyme protein brings about the correct orientation of the species for a productive collision; bringing about a higher concentration and larger rate.
Enzymes function perfectly at the “diffusion control limit”. This is what enables enzymes to provide simultaneous acid-base catalysis.
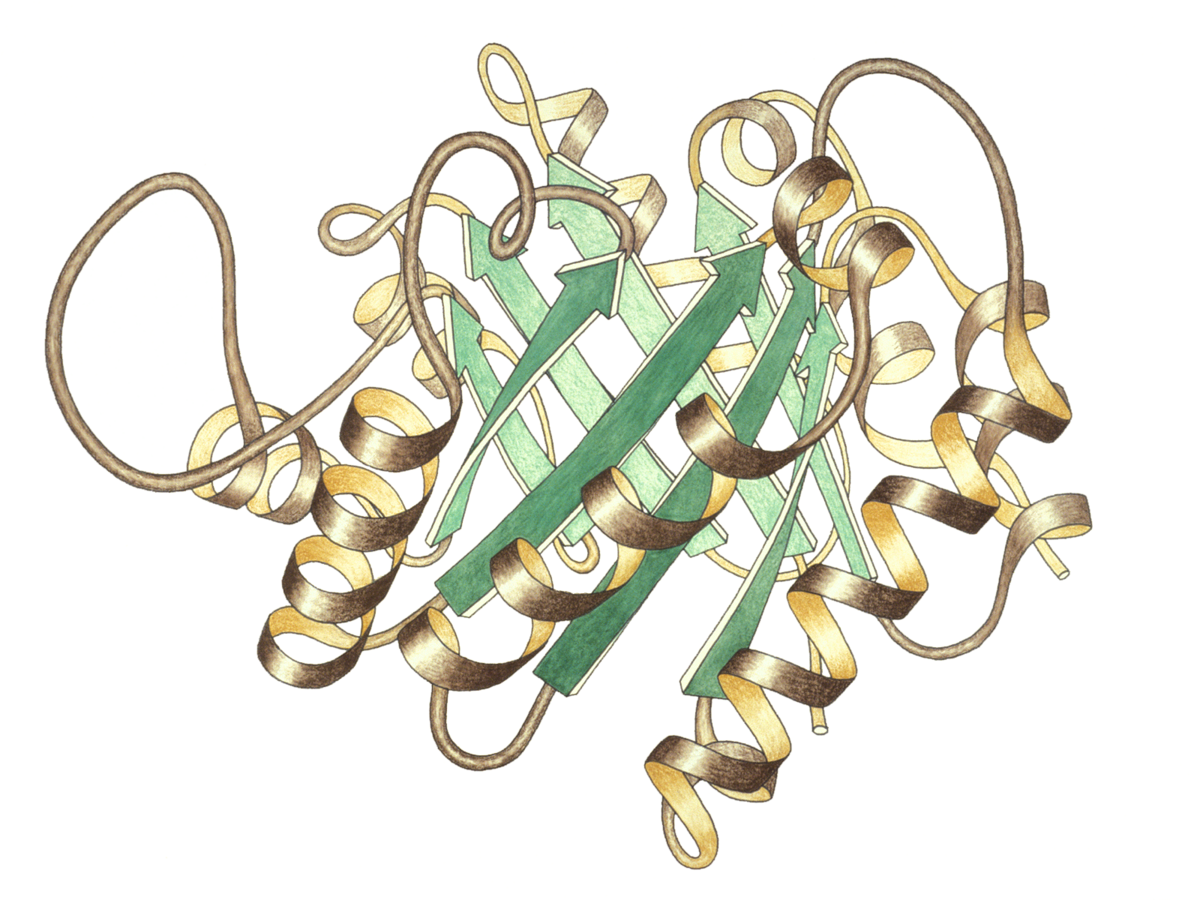
Triosephosphate isomerase (TIM) is the “perfect enzyme”. It is involved in glucose metabolism, and is present in high concentrations in muscle tissue, where it acts to generate ATP rapidly.
In glucose metabolism, glucose is broken down to the two substrates: glyceraldehyde-3-phosphate (G3P) and dihydroxyacetone phosphate (DHAP). This is an energetically unfavourable process, as it is inefficient and consumes ATP.
G3P is then converted to pyruvate, producing 2 ATP molecules and 1 NADH for each 3 carbon unit. The rate of this reaction, however, depends on the G3P concentration.
Through an equilibrium between G3P and DHAP in muscle tissues, TIM efficiently catalyses storage in a diffusion controlled conversion. This allows twenty-three times the amount of energy precursor than usual to be stored.
The equilibrium functions at a rate determined by which the substrates can diffuse through the reaction medium to get to the enzyme.
TIM is so perfect that when artificially mutated, it mutates back to perfection, with a catalytic number of 10^8 – 10^9/M/s.
The mechanism for the reaction catalysed by TIM involves the formation of an enediol intermediate. The catalytic residues glutamate and histidine are involved in general acid-base catalysis.
General acid-base catalysis is involved in a majority of enzymatic reactions, wherein the side chains of various amino acids act as general acids or general bases. General acid-base catalysis involves a molecules besides water that acts as a proton donor or acceptor during the enzymatic reaction. It facilitates a reaction by stabilising the charges in the intermediate state, through the use of an acid or base.
Nucleophilic and electrophilic groups are activated as a result of the acid/base, and causes the reaction to proceed.
An example of acid-base catalysis is peptide hydrolysis by chymotrypsin.

Chymotrypsin is involved in cleavage of amide bonds in peptides. It hydrolyses the peptide bond which connects the carboxyl group of one amino acid to the amino group of another. Key parts of the enzyme include serine, aspartic acid and histidine residues. The enzymatic reaction occurs in a step-wise process, generating a catalytic triad to improve the nucleophilic properties of serine and water.
Chymotrypsin uses a histidine residue and aspartic acid as a base catalyst. The histidine-aspartate deprotonate serine to increase its nuclephilicity. The serine then attacks the substrate’s carbonyl carbon, forming a tetrahedral intermediate. Then, an acyl group bounds to an intermediate, forming an acyl-enzyme intermediate. One product diffuses away at this time.
In carbonic anhydrase, the histidine residue helps the removal of hydrogen ion from the water molecule to generate OH- and strengthen its nucleophilic property. Once done, the water molecule attacks the acyl-enzyme, forming another tetrahedral intermediate, forming another product which diffuses away.
An oxyanion hole stabilises the tetrahedral intermediate anion formed during proteolysis and protects substrate’s negatively charged oxygen from water molecules. It stabilises the tetrahedral intermediate in chymotrypsin.
Enzymes can also attach to substrates “poised to strike”. Upon binding to a substrate, enzymes use binding energy to distort or strain the molecule on binding towards the transition state for the reaction, increasing energetic favourability.
Enzymes also work through binding in a reactive conformation. For example, phenylalanine ammonia lyase catalyses the the elimination of ammonia, and conversion of phenylalanine to cinnamic acid; putting phenylalaline amino acid into the food cycle. This reaction occurs in an “anti-configuration” environment as the hydrogen and amino group are lost, and henceforth biases the reaction.
